Exceptional musical talents and abilities
Comprehensive musician data pool from cross-sectional and longitudinal studies
Based on a large, cross-project dataset that includes both amateur and professional musicians, we have been able to demonstrate a considerable variability in individual neuro-auditory profiles. In the future, targeted meta-analyses will be conducted using our dataset, which consists of approximately 1,200 musician records. This data pool includes one-time measurements of auditory perception, neuroanatomy (MRI), and/or neurophysiology (MEG) (cross-sectional), as well as longitudinal data collected over extended periods of time. Due to the large sample size, we can create targeted subsamples based on variables such as age (~15 to 85 years), profession, exceptional auditory abilities and deficits, etc. So far, we have examined the specific abilities, talents, and preferences of children and adolescents who play music, as well as adult amateur and professional musicians across various instruments and vocal ranges, in relation to the underlying neuroanatomical and neurophysiological characteristics.
Our methodological innovations for the neuroscientific investigation of exceptional musical abilities
To analyze neuroanatomical and neurofunctional (fMRI) data, we developed specialized evaluation protocols based on the Brain Voyager QX software (Prof. Rainer Goebel, Brain Innovation, Maastricht) and Brain Electromagnetic Source Analysis (BESA GmbH, Prof. M. Scherg, Gräfelfing). These protocols enable individual segmentation, 3D reconstruction, morphometry, cortical thickness analysis, as well as the activation of auditory subareas and source analysis of auditory evoked fields (Schneider et al., 2002, 2005; Seither-Preisler et al., 2014; Benner et al., 2017, 2023; Turker et al., 2017; Zoellner et al., 2019). The methods have since been standardized and were awarded for their innovative potential (Brain Voyager Design Award, 2006). Although originally developed to study the auditory cortex, these methods are applicable to the entire brain, offering fascinating new possibilities for analyzing individual characteristics. Not only do they allow for group-specific comparisons, but they also enable the analysis of neural networks, facilitating the study of multisensory processing in addition to auditory functions.
Neuro-auditory profiles of musicians
A key aspect of our research focuses on the huge interindividual variability in basic auditory processing and pattern recognition. For instance, the frequency discrimination threshold - the ability to differentiate the pitch of two sequentially played tones - ranges from typically 2-3 semitones in children with dyslexia to less than 1 cent (< 1/100 of a semitone) in exceptionally gifted musicians (Schneider et al., 2018).
We are particularly interested in how such auditory abilities are related to musical competencies and whether these are better explained by stable neuromarkers in the brain (innate aptitude) or by musical training (learning-induced plasticity); see research area "Development of auditory and musical abilities". Comparing cross-sectional and longitudinal data, as well as examining neuro-auditory profiles of individuals with auditory processing disorders, normal-hearing amateur musicians, and various instrumentalists and singers, provides valuable insights in this regard. Based on genetic studies and our own neuroscientific findings, we hypothesize that both nature and nurture significantly contribute to the trait of "musicality," with genetics seemingly having a slightly greater influence (Schneider, P. & Seither-Preisler, A., 2015). This has important implications for music education and therapeutic practice. On the one hand, it involves identifying and fostering exceptional musical talents. On the other hand, understanding neuroplastic potential helps to better assess the likelihood of success for musical training programs, as well as music and auditory therapies for individuals with auditory processing disorders.
Our studies have shown that the neuroanatomical features of the auditory cortex differ greatly between individuals but remain largely stable within individuals from childhood to adulthood. Figure 1 illustrates the anatomical variability of the auditory cortex subareas (Heschl's gyrus, planum temporale, anterior superior temporal gyrus) in five individuals, showing substantial differences in size, shape, and right-left asymmetry (Schneider et al., 2018). These differences also have a direct impact on the auditory activation patterns of these areas (Wengenroth et al., 2014).
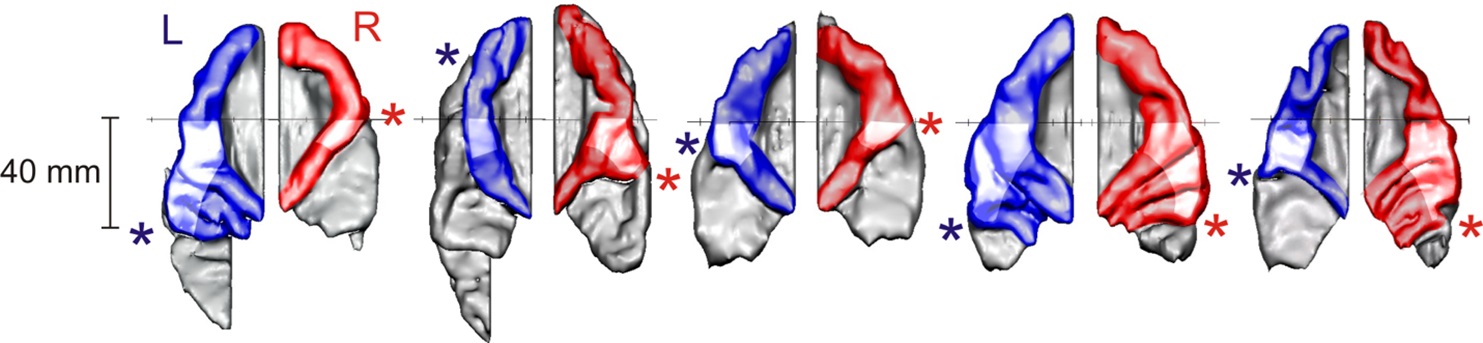
Five individual 3D reconstructions of auditory cortices; from left to right: drummer (25 y.o.), musically gifted child with dyslexia (8 y.o.), non-musician (9 y.o.), highly gifted amateur musician (48 y.o.), concert organist (85 y.o.). Color-coded (blue: left, red: right): Heschl's gyri at the center of the auditory cortex; gray: planum temporale. * denotes the lateral end of the first complete Heschl sulcus, which separates Heschl's gyri, including potential duplications, from the posterior planum temporale.
Our long-term research demonstrates a direct correlation between subjective auditory perception and neuroanatomical and neurofunctional features (Schneider et al., 2002, 2005, 2005a, 2023; Seither-Preisler et al., 2014).
Regarding the rare ability to immediately identify and name the pitch of a heard sound ("absolute pitch"), we were the first to identify a right-hemispheric neural network representing the qualitative sensory experience. Additionally, we discovered co-activation of Broca's area in the left hemisphere, responsible for "labeling" the perceived pitches into musical pitch categories (Wengenroth & Schneider, 2014).
There are also significant individual differences in the perception of harmonic sound spectra, which are typical in singing and produced by most musical instruments. Harmonic sounds are characterized by their temporal periodicity (fundamental pitch) and their frequency spectrum (overtones, which contribute to timbre). The aspect that dominates subjective perception largely depends on individual neuroanatomical characteristics. Individuals who predominantly perceive the fundamental pitch tend to have a higher volume of gray matter in the left Heschl's gyrus, whereas those who focus more on overtones have a larger volume in the right Heschl's gyrus. This is reflected in the auditory evoked responses generated in these areas (Schneider et al., 2005). These individual differences also correspond to instrument preference (Schneider & Wengenroth, 2009). Fundamental-pitch listeners often choose instruments that require high temporal precision, such as percussion, guitar, piano, or trumpet. Overtone listeners, on the other hand, tend to prefer instruments where subtle nuances in timbre are essential, while temporal accentuation plays a lesser role, such as the organ, oboe, clarinet, horn, or cello. This pattern aligns with the fact that the left auditory cortex is specialized for the sequential processing of rapidly fluctuating information, while the right auditory cortex is specialized for the parallel analysis of spectral components (Schneider & Seither-Preisler, 2022, 2023). This dichotomy is even reflected in the seating arrangement of a symphony orchestra, where percussive and solo instruments, often played rapidly and dynamically, are found on the left, while instruments rich in timbre for accompaniment are positioned on the right. This pattern is mirrored in the brains of the respective musicians, as observed by Peter Schneider in studies of the Mannheim National Orchestra and the Royal Liverpool Philharmonic Orchestra (RLPO) (Schneider et al., 2005a; see Fig. 2).
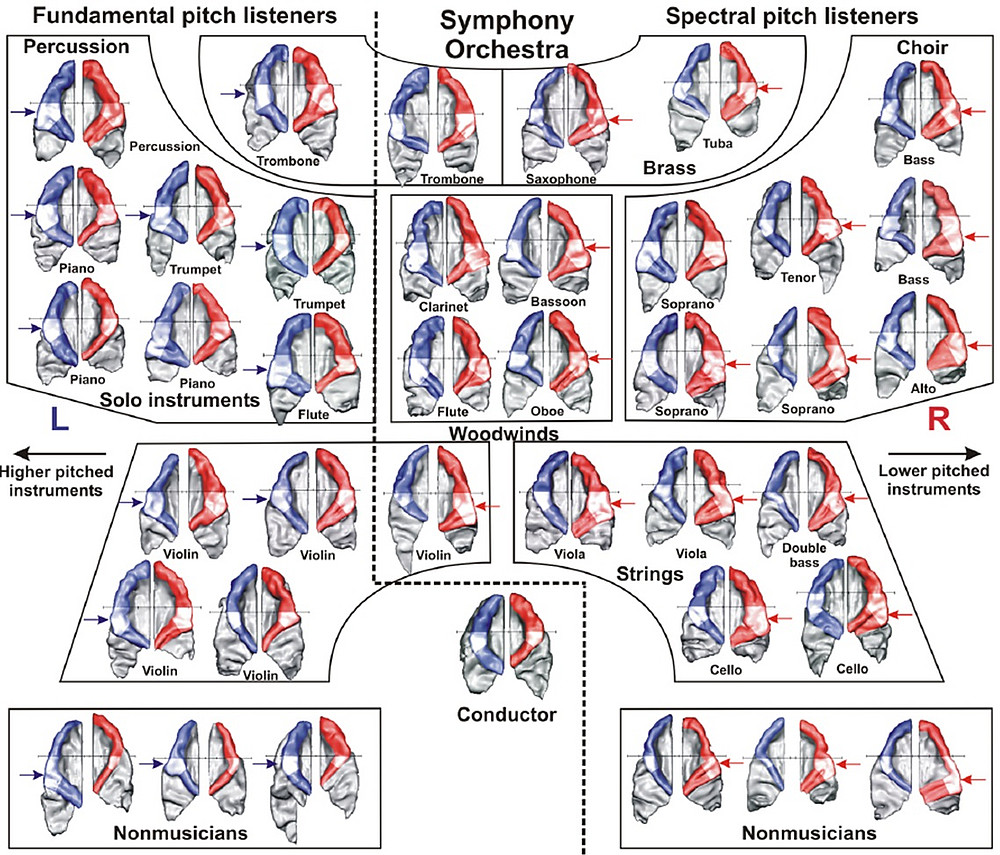
Auditory cortices of selected musicians from the Mannheim National Orchestra and the Royal Liverpool Philharmonic Orchestra, indicating the instruments played and their position in the orchestra. The volume of the anterolateral part of Heschl's transverse convolutions in the right and left hemisphere, which is essential for sound processing, is marked in white.
The data obtained using various neuroscientific and psychoacoustic methods can be summarized into an individual neuro-auditory profile. Figure 3 illustrates such a profile using the example of a conductor.
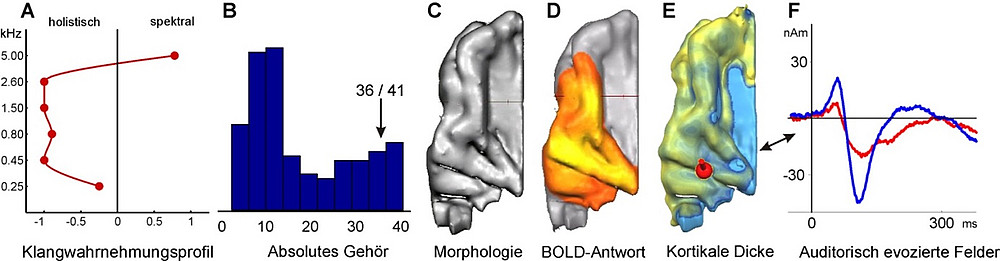
Fig. 3:
Neuro-auditory profile of a female conductor. (A) Sound perception profile (overtone-fundamental test; Schneider et al., 2005), (B) Gradual manifestation of absolute pitch (Wengenroth et al., 2014), (C) Morphology of the auditory cortex, (D) Functional BOLD activation while listening to sounds, (E) Cortical thickness and localization of the MEG dipole (red sphere), (F) Time dependency of auditory evoked responses in the right (red line) and left (blue line) hemispheres while listening to instrumental sounds. These individual characteristics provide insights into which musical instrument, music style, and educational learning strategy may be particularly well-suited.
To date, it remains unexplored to what extent such anatomical and functional variability might also be found in other areas of the brain. Our pilot studies clearly point in this direction. Figure 4 provides an example, showing various brain anatomical structures of a musically and cognitively highly gifted adolescent. Correspondences can be observed between the individual gyrification of visual subareas (Fig. 3, A), the hand-knob area in the precentral gyrus (B), and the auditory cortex (C). An overarching pattern in this case is the presence of multiple gyrifications.
Fig. 4:
Examples of an individual visual-motor-auditory profile of a highly gifted adolescent. (A) Segmented visual cortex; the unfolded area between the calcarine sulcus and parieto-occipital sulcus is color-coded. (B) Segmented right hand-knob, viewed from behind, showing the precentral gyrus. (C) Heschl’s gyri, viewed from above.
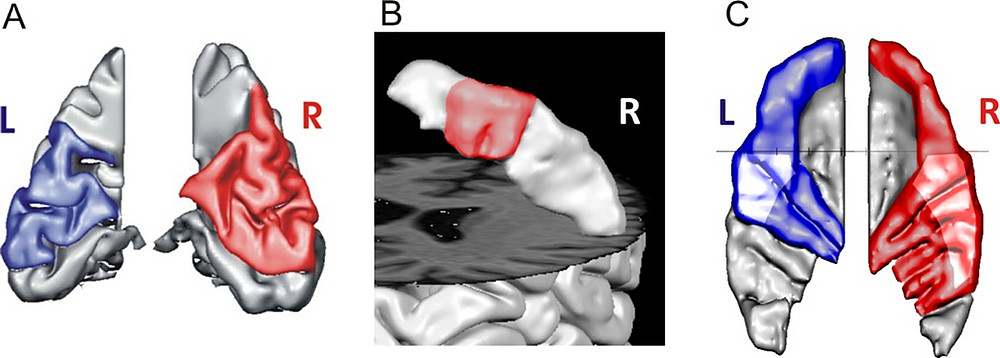
This raises the question of whether these are isolated cases or if there are typical patterns where the features of auditory, motor, linguistic, prefrontal, and/or visual areas correspond with each other. Are there overarching architectural or phenotypological characteristics in the structure of the human brain, and if so, how do these relate to specific abilities, exceptional talents, or cognitive deficits? Do certain "morphological profiles" exist that indicate high linguistic, visuospatial, or numerical-mathematical potentials?
Our previous research has already revealed a neuroanatomical overlap between musical and linguistic functions, as well as related exceptional talents. Specifically, we found that the volume of gray matter in the right Heschl's gyrus can be considered a neuromarker for both musical (Seither-Preisler et al., 2014) and linguistic (Turker et al., 2017) aptitude. For more on the topic of music and language, click here. Whether such shared neurological resources also exist for other cognitive domains will be the subject of future research.
References
Benner, J., Reinhardt, J., Christiner, M., Wengenroth, M., Stippich, C., Schneider, P., & Blatow, M. (2023). Temporal hierarchy of cortical responses reflects core-belt-parabelt organization of auditory cortex in musicians. Cerebral Cortex, 33(11), 7044-7060. https://dx.doi.org/10.1093/cercor/bhad020
Schneider, P., Engelmann, D., Groß, C., Bernhofs, V., Hofmann, E., Christiner, M., […] & Seither-Preisler, A. (2023). Neuroanatomical disposition, natural development, and training-induced plasticity of the human auditory system from childhood to adulthood: a 12-year study in musicians and nonmusicians. Journal of Neuroscience, 43(37), 6430-6446. https://doi.org/10.1523/JNEUROSCI.0274-23.2023
Schneider, P., Groß, C., Bernhofs, V., Christiner, M., Benner, J., Turker, S., […] & Seither‐Preisler, A. (2022). Short‐term plasticity of neuro‐auditory processing induced by musical active listening training. Annals of the New York Academy of Sciences, 1517(1), 176-190. https://doi.org/10.1111/nyas.14899
Zoellner, S., Benner, J., Zeidler, B., Seither‐Preisler, A., Christiner, M., Seitz, A., […] & Schneider, P. (2019). Reduced cortical thickness in Heschl's gyrus as an in vivo marker for human primary auditory cortex. Human brain mapping, 40(4), 1139-1154. https://doi.org/10.1002/hbm.24434
Schneider, P., Benner, J., Zeidler, B., Christiner, M., Seither-Preisler, A., & Engelmann, D. (2018). Audio- und Neuroplastizität der Klangwahrnehmung. Akustik Journal, 2, 16-30. https://www.dega-akustik.de/fileadmin/dega-akustik.de/publikationen/akustik-journal/18-02/akustik_journal_2018_02_online_artikel2.pdf
Benner, J., Wengenroth, M., Reinhardt, J., Stippich, C., Schneider, P., & Blatow, M. (2017). Prevalence and function of Heschl’s gyrus morphotypes in musicians. Brain Structure and Function, 222, 3587-3603. https://doi.org/10.1007/s00429-017-1419-x
Turker, S., Reiterer, S. M., Seither-Preisler, A., & Schneider, P. (2017). “When music speaks”: Auditory cortex morphology as a neuroanatomical marker of language aptitude and musicality. Frontiers in Psychology, 8, 2096. https://doi.org/10.3389/fpsyg.2017.02096
Schneider, P., & Seither-Preisler, A. (2015). AMseL-Neurokognitive Korrelate von JeKi-bezogenem und außerschulischem Musizieren. In U. Kranefeld (Ed.), Instrumentalunterricht in der Grundschule. Prozess- und Wirkungsanalysen zum Programm Jedem Kind ein Instrument (pp. 19-48). BMBF.
Seither-Preisler, A., Parncutt, R., & Schneider, P. (2014). Size and synchronization of auditory cortex promotes musical, literacy, and attentional skills in children. Journal of Neuroscience, 34(33), 10937-10949. https://doi.org/10.1523/jneurosci.5315-13.2014
Wengenroth, M., Blatow, M., Heinecke, A., Reinhardt, J., Stippich, C., Hofmann, E., & Schneider, P. (2014). Increased volume and function of right auditory cortex as a marker for absolute pitch. Cerebral Cortex, 24(5), 1127-1137. https://dx.doi.org/10.1093/cercor/bhs391
Schneider, P., & Wengenroth, M. (2009). The neural basis of individual holistic and spectral sound perception. Contemporary music review, 28(3), 315-328. https://dx.doi.org/10.1080/07494460903404402
Schneider, P., Sluming, V., Roberts, N., Scherg, M., Goebel, R., Specht, H. J., […] & Rupp, A. (2005a). Structural and functional asymmetry of lateral Heschl's gyrus reflects pitch perception preference. Nature Neuroscience, 8(9), 1241-1247. https://doi.org/10.1038/nn1530
Schneider, P., Sluming, V., Roberts, N., Bleeck, S., & Rupp, A. (2005b). Structural, functional, and perceptual differences in Heschl's gyrus and musical instrument preference. Annals of the New York Academy of Sciences, 1060(1), 387-394. https://dx.doi.org/10.1196/annals.1360.033
Schneider, P., Scherg, M., Dosch, H. G., Specht, H. J., Gutschalk, A., & Rupp, A. (2002). Morphology of Heschl's gyrus reflects enhanced activation in the auditory cortex of musicians. Nature Neuroscience, 5(7), 688-694. https://doi.org/10.1038/nn871